Predicting fatigue crack initiation in engineering alloys is a long-standing challenge. It requires model validation via precise quantitative comparison to measurements of deformation gradients across many length scales, particularly in the vicinity of stress concentration features. The comprehensive work of Gustafson and collaborators reported here employs four different high-energy x-ray techniques at two different synchrotrons, combined with advanced crystal plasticity modeling, to characterize deformation gradients near twin boundaries that can lead to fatigue failure in Ni-based superalloys. High energy x-ray diffraction microscopy in both the near-field and far-field (NF-HEDM and FF-HEDM) were employed at CHESS to characterize the microstructure and monitor grain average strain evolution during cyclic loading applied by the RAMS2 load frame. These measurements identified a single microscopic grain within the larger sample volume with a desired orientation and significant plasticity localization. Informed by HEDM, this grain was located and cut from the larger sample via focused ion beam milling, and further characterized via diffraction tomography and dark-field microscopy at the European Synchrotron Radiation Facility. The resulting data gives holistic quantitative insight into fatigue-relevant deformation gradients from millimeter to micron scales.
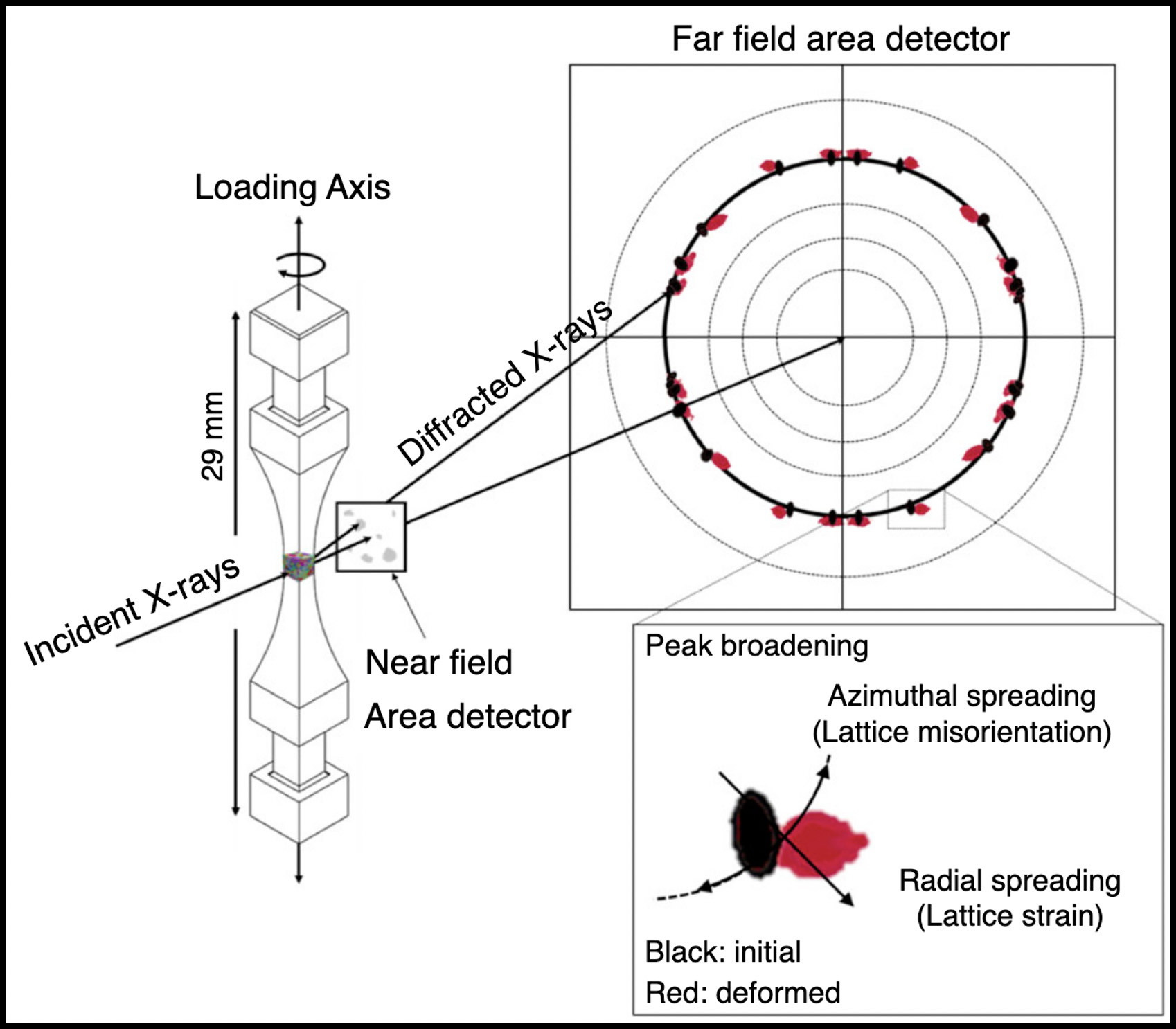
Importance
The sub-surface region of the studied grain, in direct proximity to the twin boundary, exhibits sharp gradients in both elastic strain and crystal lattice reorientation. The plasticity model, confirmed by the experimental data, identified the twin boundary as the direct cause of these large gradients. These results provide insight into the development of the micro-mechanical fields prior to fatigue crack initiation and could help define fatigue failure criteria. This ongoing research will validate the next generation of advanced, multi-scale mechanical performance models, promising more accurate lifetime prediction of structural components with reduced uncertainty.
Need for CHEXS Experimental Capabilities
The combination of near-field and far-field high-energy x-ray diffraction microscopy measurements of a full sample gauge volume, while performing cyclic loading in tension/compression, is a core capability of the structural materials program at the FAST beamline at CHEXS. The RAMS2 load frame used in this study is one of several specialized and unique sample environments available to users at FAST. The computational resources required for co-analysis of different diffraction microscopy techniques are also supported by CHEXS.
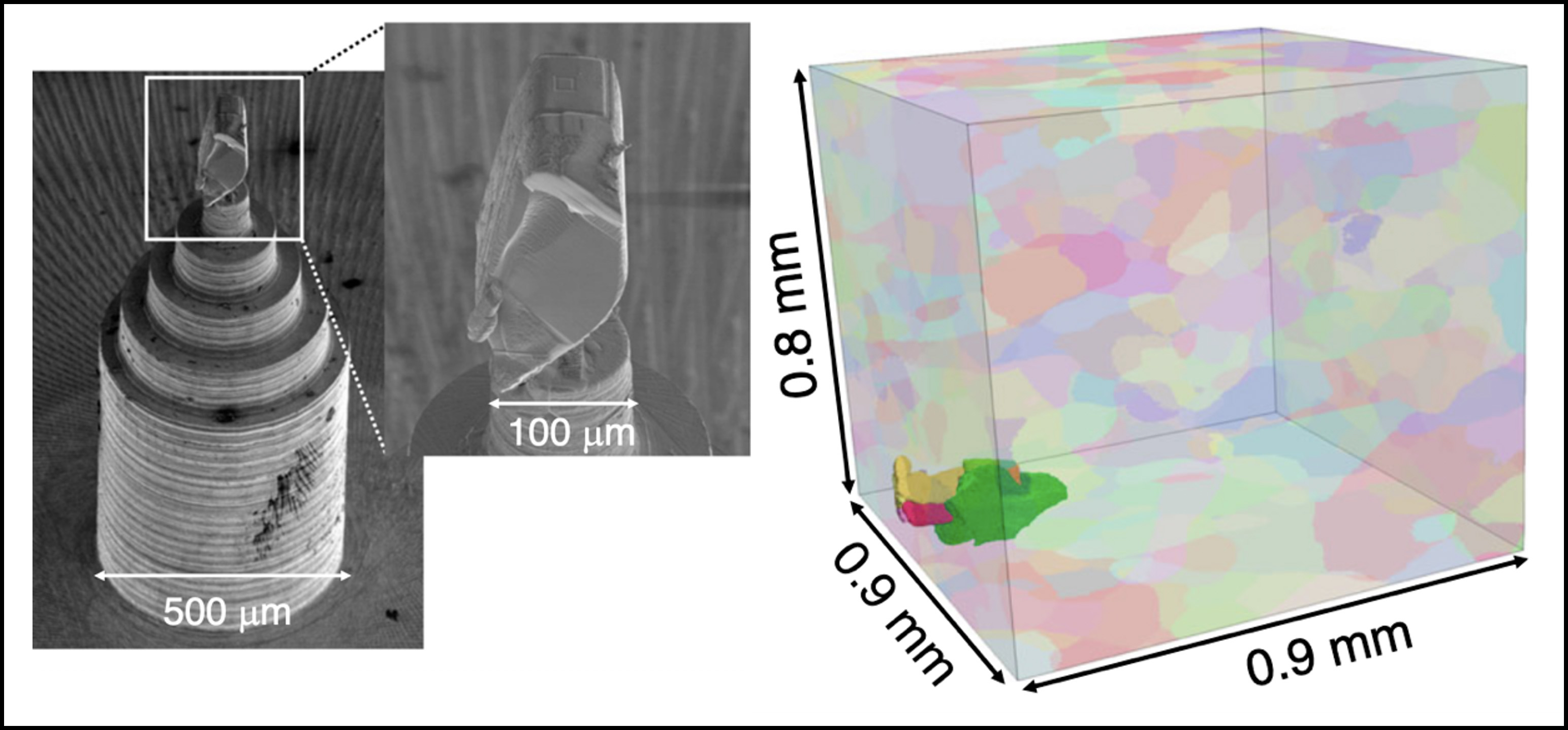
Funding
This work was supported by the National Science Foundation under CMMI 16-51956. Travel support was provided by the Defense Advanced Research Projects Agency under N66001-14-1-4041 and HR0011-12-C-0037. In situ high energy X-ray microscopy was performed at the Cornell High Energy Synchrotron Source (supported by the National Science Foundation under DMR-1829070). Authors acknowledge support from the Materials & Manufacturing Directorate and the Air Force Office of Scientific Research of the U.S. Air Force Research Laboratory.
Reference Sven Gustafson, Wolfgang Ludwig, Paul Shade, Diwakar Naragani, Darren Pagan, Phil Cook, Can Yildirim, Carsten Detlefs & Michael D. Sangid. Quantifying microscale drivers for fatigue failure via coupled synchrotron X-ray characterization and simulations.
Nature Communications 11, 3189 (2020). https://doi.org/10.1038/s41467-020-16894-2