Numerous construction materials are utilized deep below the earth’s surface and these materials must maintain their structural integrity for both personal and environmental safety. For this reason, there is a major push to develop new structural materials that are sustainably produced while also ensuring infinite lifetimes. In order to deploy these new materials, researchers must understand how they perform in the high pressure environments that exist deep below the ground. This is because materials used for geotechnical endeavors such as oil and gas wells, pilings, and tunneling exhibit significantly different mechanical properties when under the intense pressures found below the earth’s surface than at the surface.
Historically, construction materials were only able to be mechanically tested at the macroscopic scale when subjected to high pressure and there was no means to probe their micromechanical responses through in-situ testing. Due to these limitations, the underlying microscopic deformation mechanisms leading to failure have been hypothesized and modeled, but these models have not been completely validated.
Validating these mechanisms and models is critical for accelerating the adoption of new material systems in the field.
CHESS is now working to enable such validations. A triaxial pressure cell has been designed and constructed for use at the Forming and Shaping Technology (FAST) beamline, part of the NSF-funded Center for High Energy X-ray Sciences (CHEXS). This pressure cell is a unique device able to apply compressive loads up to 2 kN in addition to hydrostatic pressures up to 100 MPa, while allowing far-field High-Energy Diffraction Microscopy experiments to be performed.
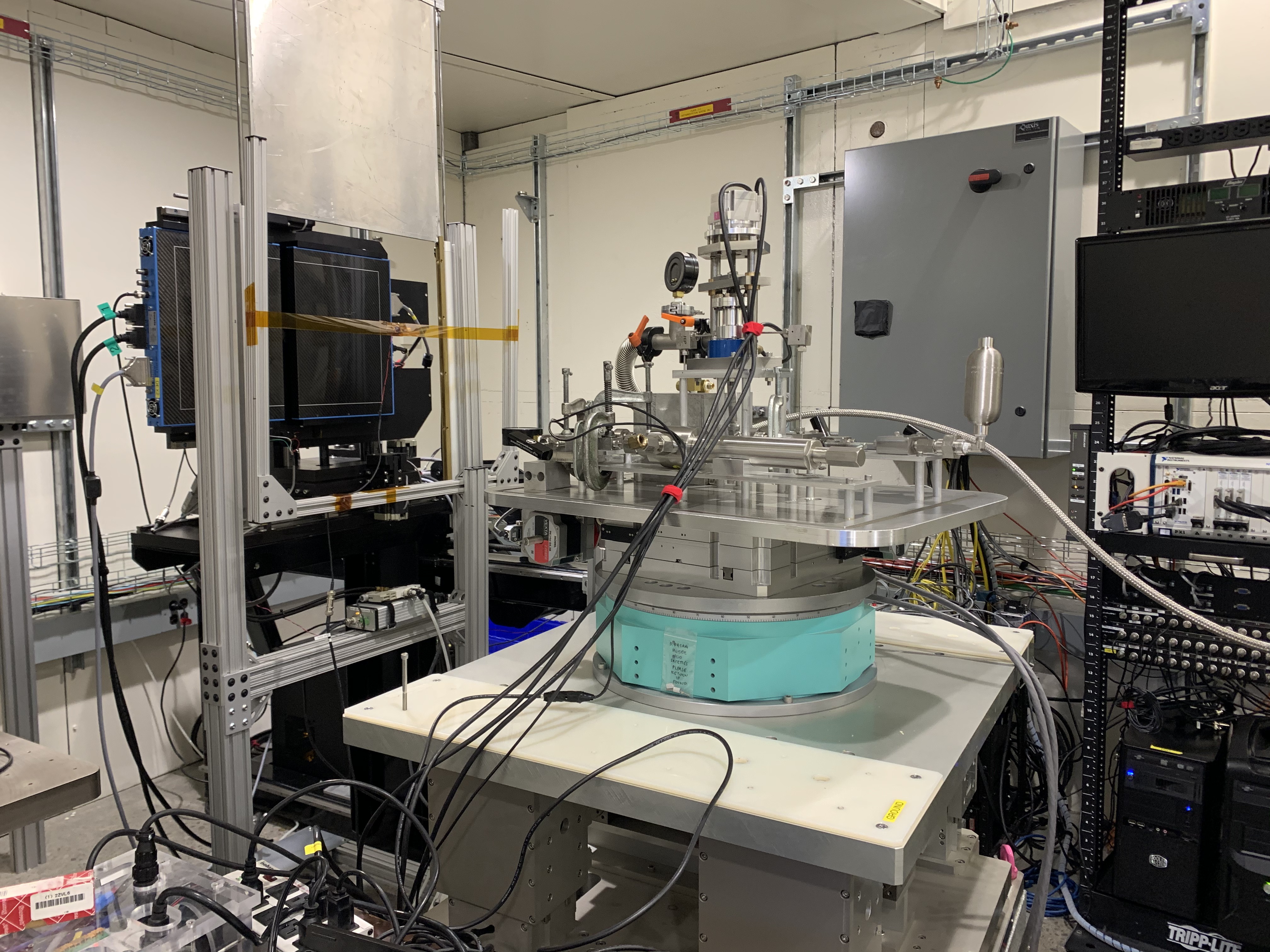
Using this device users can explore the micromechanical deformation behavior of cementitious materials, engineering alloys, and geomaterials in pressure environments simulating up to ~25km below the surface. More specifically, users can measure the elastic strains, lattice orientations, and centroids of individual crystals during the application of adjustable macroscopic triaxial stress states. With this capability, users can explore the micromechanical origins of macroscopic properties.
Initial testing of the device was performed at the FAST beamline in October, 2019. Hydrostatic pressures up to 75 MPa were applied to quartz sand, and then compressive loads were applied to the specimen. Figure 2 shows an example of data gathered using the device, with the von Mises equivalent stresses in individual sand grains plotted against their position in the specimen as compressive load and pressure are applied. With this spatial resolution, users can begin to pinpoint the origin of failure at the microscale.
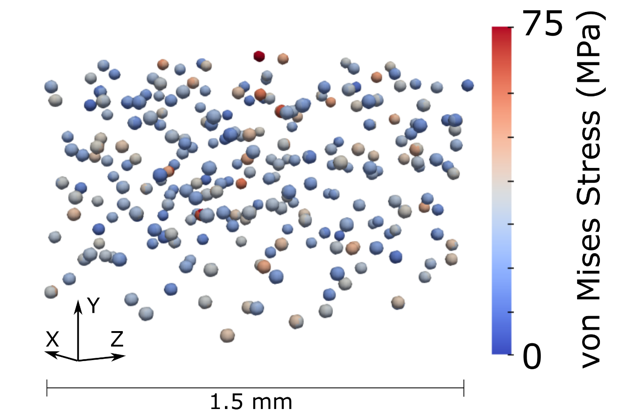
The development of this pressure cell has been a multi-year effort at CHESS, with the preliminary design completed by Dane Brown as a Mechanical Engineering senior design project. The project has since been continued by Sriramya Nair, currently a faculty member in the Civil and Environmental Engineering Department, and Connor Horn, currently completing his senior year in the Applied Physics Department.
The triaxial pressure cell will be available to CHEXS users starting February, 2020 and can be included in FAST (Beamline 3A) proposals.